REU: Undergraduate Research Opportunities in Biomedical Engineering Devices
Design and innovate with novel biomedical engineering device technologies!
Pending funding approval.
Description
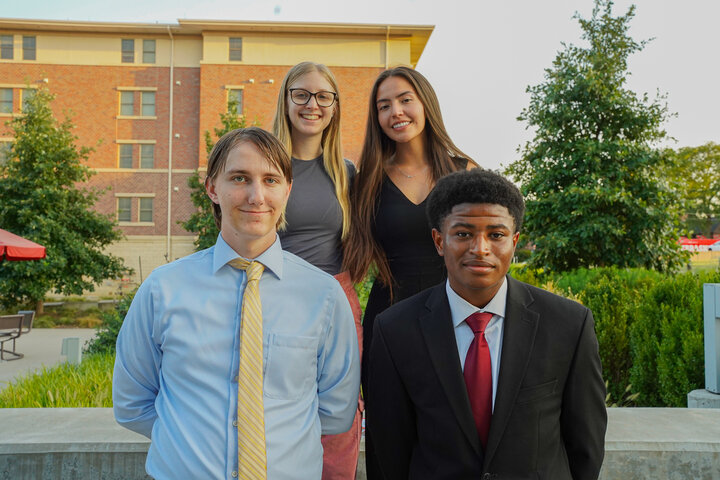
The Biomedical Engineering REU is designed to provide independent research experience for undergraduate students, broaden participant knowledge of opportunities in academia, industry and national laboratories, and introduce participants to interdisciplinary research in biomedical devices.
The goal of every medical practitioner is to improve quality of life for patients. Biomedical engineering and devices are instrumental in achieving this. The primary focus in each summer research project is biomedical devices designed to enhance medical care through science and engineering, with emphasis in two areas: (1) devices for diagnostics and sensing and (2) devices for therapeutics and intervention.
All projects are designed to be completed during the 10 week program and are a part of a faculty mentor's current research. This allows the student to be involved in many aspects of research, including design, analysis, simulation, and implementation of a biomedical device.
Students are also extensively involved in lab activities, such as weekly lab meetings. Research results are presented during lab meetings throughout the summer and at the end-of-summer in the Summer Research Symposium poster session. Lab members, especially graduate students and postdoctoral associates, are active with summer program research.
See the list below for associated mentors and projects.
Benefits
- Competitive stipend: $7,000
- Suite-style room and meal plan
- Travel expenses to and from Lincoln
- Campus parking and/or bus pass
- Full access to the Campus Recreation Center and campus library system
- Wireless internet access
Learn more about academic and financial benefits.
Events
- Department seminars and presentations
- Professional development workshops (e.g., applying to graduate school, taking the GRE)
- Welcome picnic
- Day trip to Omaha's Henry Doorly Zoo and Aquarium
- Outdoor adventures
- Research symposium
Questions about this program?
Please direct any questions related to this program to:
Eric Markvicka: 402-472-1617 • eric.markvicka@unl.edu
Assistant Professor > Mechanical & Materials Engineering
Who Should Apply
Related Fields of Study
- Physics
- Biological Sciences
- Any engineering major with interest in medical applications
- Any science major with interest in medical applications
This program encourages applications from students with junior or senior standing.
Eligibility
Participation in the Nebraska Summer Research Program is limited to students who meet the following criteria:
- U.S. Citizen or Permanent Resident
- Current undergraduate with at least one semester of coursework remaining before obtaining a bachelor's degree
See Eligibility for more information.
How to apply
Follow the application steps to submit the following materials.
Mentors and Projects
MENTORS | PROJECTS |
---|---|
Dr. Greg Bashford, Dr. Eric MarkvickaBIOLOGICAL SYSTEMS ENGINEERING, MECHANICAL & MATERIALS ENGINEERING | Design and development of a wearable ultrasound patch for measuring cerebral blood flow Ultrasound is a safe, noninvasive method of measuring blood flow in the body, and transcranial Doppler (TCD) is a special ultrasound mode that measures blood flow in cerebral arteries deep in the brain [5]. TCD may be used as an inexpensive, portable method to measure the brain’s response to stimuli since neural activity is correlated with blood flow in the cerebral cortex. However, it is currently challenging to continuously monitor brain response. In this context, skin-mounted wearable ultrasound devices emerge as a promising alternative, poised to facilitate prolonged, uninterrupted patient monitoring [6-9]. This project will create a wearable Doppler ultrasound patch for continuous measurement of cerebral blood flow (PI Markvicka). This project will also assess the use of a new data index based on systems theory (relaxation time; Co-PI Bashford). This is a team-based REU project where two students will work jointly with Dr. Bashford and Dr. Markvicka. The REU student working with Dr. Bashford will immediately be taught to scan subjects; by the first week’s end, they will be able to measure blood flow in a fellow student using a handheld TCD ultrasound device. By week four, the student will have participated in experiments scanning 10 human subjects designed to test the index described above. The other student working with Dr. Markvicka will be taught advanced manufacturing approaches for creating soft, skin-like electronic devices including injection molding, soft lithography, and UV laser micromachining and material characterization techniques to measure elastic modulus, maximum elongation, and acoustic impedance. By week four, the student will have created multiple prototypes of a wearable Doppler ultrasound device consisting of a two-layer circuit with piezoelectric transducer and appropriate matching layer for acoustic impedance matching. During the next three weeks, the students will work together to characterize the wearable ultrasound device and measure blood flow in an ultrasound flow phantom. The students will use an iterative approach to assure the device is capable of continuously measuring cerebral blood flow. In the final third of the summer, the student will be encouraged to develop (and present to Dr. Bashford’s and Dr. Markvicka’s lab) their own ideas about what the next steps would be if they were the project lead. Each student will prepare a poster for the UNL Research Symposium, and if results warrant, draft a paper for peer-reviewed publication. |
Dr. Nicole IversonBIOLOGICAL SYSTEMS ENGINEERING | Carbon Nanotube Sensor Platform to Quantify Nitric Oxide and Hydrogen Peroxide Levels in Inflammatory Diseases Reactive oxygen and nitrogen species have been shown to be important factors in the progression of many diseases, ranging from autoimmune disease to cancer, but until recently there has been a lack in the ability of researchers to study nitric oxide and hydrogen peroxide (key reactive nitrogen and oxygen species) in real time. By wrapping carbon nanotubes with a specific DNA strand, a real time sensor for nitric oxide or hydrogen peroxide can be made. The student involved in this project will gain hands-on experience in BME research, with a focus on nanotechnology, sensors, and the engineering design process. During the first week, the student working with Dr. Iverson will start building and characterizing carbon nanotube sensor platforms. As the student becomes more comfortable with the project, they will design experiments and interpret data independently, discussing their results and brainstorming about future work with Dr. Iverson and the other lab members. In parallel, the student will be introduced to primary literature searches, sterile culture technique, and the importance of nitric oxide and hydrogen peroxide in cell survival and disease progression. The REU student will finish the summer with an understanding of experimental design, data analysis, and interpretation of results as well as having been exposed to cutting-edge research. |
Dr. Forrest KievitBIOLOGICAL SYSTEMS ENGINEERING | Nanoparticle-Mediated Sensitization of Pediatric Brain Tumors to Radiotherapy The field of nanomedicine offers the potential to improve the understanding and treatment of many disease processes by allowing researchers and clinicians the ability to deliver treatments to specific areas of the body, image where the treatments are going in real-time, and track responses. Therefore, the development of multifunctional nanoparticles has garnered significant attention especially for improving delivery into the brain for neurological diseases including traumatic brain injury, brain cancer, and dementia for which there is a significant lack of effective treatment options. These nanoparticles typically consist of a small core that acts as a scaffold to carry imaging agents for tracking nanoparticle localization in the body through various imaging modalities such as magnetic resonance and fluorescence imaging, therapeutic moieties for treatment, and targeting agents for binding cell surface receptors expressed in target tissue. However, the translation of nanomedicine into clinical use has been hindered by complicated nanoparticle designs that make reproducible synthesis and scale-up difficult. Therefore, a goal of the Kievit lab is to develop simplified synthesis strategies for multifunctional nanoparticles to improve their translatability. The student working with Dr. Kievit will be introduced to nanoparticle drug delivery vehicles as well as various mechanisms to gain entry into the brain through shared relevant literature before they arrive on campus. By the end of the first week on campus, the student will be trained on the nanoparticle synthesis and characterization strategies for the type of nanoparticles they will be working with such as magnetic and polymeric nanoparticles that can be imaged using both MRI and fluorescence imaging. Once this is complete, the student should be able to formulate a hypothesis on how to achieve the project goal as well as methods to test this hypothesis. Advanced students will also learn how to image the nanoparticles using our 9.4T MRI and fluorescence microscopes. During the middle of the summer, the student will use their developed skills to test their hypothesis by generating and interpreting characterization and imaging results. During the final third of the summer the student will reformulate their hypothesis based on their preliminary results and use their methods to re-test this hypothesis (search and re-search!). During this project, the student is expected to gain an understanding of the limitations of neurological disease treatment and how nanoparticle engineering strategies can be used to overcome these limitations. The student will get hands-on experience in nanoparticle synthesis strategies, characterization methods, and use of different imaging systems. The student will also get the experience of processing, analyzing, and interpreting experimental data, as well as data presentation in written and oral form. |
Dr. Fanben Meng, Dr. Wei Niu, Dr. Yinsheng GuoMECHANICAL & MATERIALS ENGINEERING, CHEMICAL & BIOMOLECULAR ENGINEERING, CHEMISTRY | Ultra-High-Throughput Microdroplet Screening for Protein Biologics Discovery Protein biologics, e.g., antibodies and enzymes, are in high demand in applications ranging from affinity reagents in biomedical research and therapy to biocatalysts for the manufacturing of small-molecule drugs. The ability to quickly identify a protein of the desirable functionality is often limited by the throughput of the screening method. Three labs across the UNL campus work collaboratively on a project to develop and apply an ultra-high-throughput microdroplet method using lab-on-chip devices. This research team includes Dr. Wei Niu in Chemical and Biomolecular Engineering Department (protein engineering), Dr. Fanben Meng in Mechanical and Materials Engineering Department (microfluidic devices), and Dr. Yinsheng Guo in Chemistry Department (optical detections). REU students on this project work as a team with individual tasks that are integrated into the overall project goal. The student in Dr. Niu’s lab focuses on the construction of protein mutant library and the characterization of potential hits obtained from the droplet screening. The student in Dr. Meng’s lab works on the fabrication of microfluidic chips and the generation of microdroplets. The student in Dr. Guo’s lab is responsible for the optical detection and the isolation of hits. The students further work together to complete successful screening experiments. The team meets weekly for project updates and troubleshooting. Besides knowledge of the biomedical research field, REU students on this project also gain valuable experiences on research collaboration and build skills needed in a team environment. |
Dr. Nitesh NamaMECHANICAL & MATERIALS ENGINEERING | Ultrasound-Propelled Microswimmers for Manipulation of Synthetic Objects within Blood Vessels The ability to move synthetic objects precisely within blood vessels can open exciting avenues for several applications in biomedicine and healthcare. Given the small size of blood vessels, an important requirement for achieving these functionalities is the ability to precisely direct a “microswimmer” in a controlled and tunable manner. The student will leverage computational approaches to devise novel acoustics-based propulsion approaches, that can enable controlled, tunable motion at microscales. The main research questions are the following: (1) What are the acoustic forces that govern the motion of a microswimmer? and (2) How can the operational parameters of the acoustic field (e.g., frequency, power) be optimized to achieve a desired motion of microswimmers to reach a specific target site? Prior to arriving on campus, the student working with Dr. Nama will be provided with relevant literature and COMSOL software training. At the end of week 1, the student will be able to able perform preliminary simulations on the forces experienced by microswimmers under acoustic actuation and integrate this data to design preliminary microswimmer trajectories. In weeks 2-4, the student will receive extensive training on MATLAB concerning basic programming and plotting techniques. This training will also enable the student to plot their results and prepare their final presentation. Subsequent three weeks will focus on studying the effects of acoustic frequency and power by conducting several simulations for different frequencies. The last three weeks will be used to compare the computational data against the available experimental data, and design and test novel microswimmer designs. |
Dr. Carl NelsonMECHANICAL & MATERIALS ENGINEERING | Robotic Technology for Next-Generation Minimally Invasive Surgery Robotic tools are becoming a standard fixture in medicine, and particularly in surgery, where they can help enhance dexterity and visualization in minimally invasive approaches. Inserted, in-vivo robots (like miniature surgeon arms inside the abdomen) can have particularly high functionality. However, the small electric motors that typically drive these surgical robots are problematic: they take up too much space for too little force and speed capability, and electrical connections multiply the possible failure modes and reduce reliability. Dr. Nelson’s lab is developing strategies for actuator integration and modularity to increase functionality and reliability of these surgical robots while dramatically lowering cost. The specific research purpose of this project is to investigate the efficacy of different actuator and linkage layouts to maximize robot functionality and reliability. The REU student will perform hands-on testing of prototype robot components (beginning in week 1) and collect data (beginning in week 2) on their performance. As (s)he gains familiarity with the project, the participant will progress to computer-aided design (by week 4) and simulation and prototype fabrication (by week 6) of his/her own original designs and/or modifications to existing designs. It is expected that along the way, (s)he will learn and apply principles of robot kinematics and dynamics, biomaterials selection, and engineering design methodology, along with somewhat lighter exposure to anatomy/physiology and computer science. |
Dr. Angela PannierBIOLOGICAL SYSTEMS ENGINEERING | DNA-Loaded Nanocarriers for Enhanced Oral Gene Delivery and Vaccination Oral gene delivery offers a promising strategy for gene-based therapy. It is non-invasive, convenient, and can be used for both local and systemic gene production. This includes treating gastrointestinal diseases and using DNA delivery for vaccination. However, nonviral methods face challenges in protecting DNA and promoting uptake by intestinal cells, resulting in low transgene expression. To address this, we are developing DNA-loaded OMV nanocarriers (DNA-OMV NCs) derived from gut bacteria. The student working with Dr. Pannier will create methods to produce DNA-OMV NCs and evaluate their abilities to protect DNA cargo, and mediate transfection. Prior to arriving on campus, the student will read relevant background literature and receive web-based training materials to expediate lab work once they arrive. During the first week, the student will begin with training on specific assays, including mammalian and cell culture and OMV isolation. Over the course of the summer they will also learn how to prepare plasmid DNA, load plasmid DNA into OMVs, and then deliver DNA-loaded OMVs to both intestinal epithelial cells and macrophages. The student will be responsible for determining and testing optimization parameters to maximize DNA loading to achieve high levels of transfection with low toxicity. These findings will guide our design of OMVs as oral gene delivery carriers. At the end of the summer the student will present their work, and have the opportunity to prepare an abstract for the BMES meeting. |
Dr. Ryan PedrigiMECHANICAL & MATERIALS ENGINEERING | Ultrasound as a Mechanotherapy for Heart Disease and Stroke Atherosclerosis is the fundamental pathology in heart disease and ischemic stroke characterized by the accumulation of fat and immune cells in the inner lining of arteries. An interesting feature of this disease is that these fatty plaques tend to form in regions of arteries that experience certain mechanical interactions between blood flow and the endothelial cells that sit at the inner lining of arteries. The ability to deliver beneficial mechanical stimuli to endothelial cells in these artery regions could be therapeutic. We are currently studying the use of ultrasound for this purpose and ultimately aim to develop a new therapy for atherosclerosis using this technology. This project will work on characterizing the biological response of endothelial cells to ultrasound. During weeks 1-2, the student working with Dr. Pedrigi will learn about ultrasound and become proficient using traditional and custom-made ultrasound transducers. During weeks 3-4, the student will become proficient with basic cell culture techniques. During weeks 5-7, the student will perform experiments with endothelial cells exposed to ultrasound and learn about immunostaining and microscopy. For the remainder of the summer, the student will evaluate the biological response of endothelial cells to a range of ultrasound regimens. |
Dr. Benjamin RigganELECTRICAL & COMPUTER ENGINEERING | Domain Adaptative Computer Vision Algorithms for Biomedical Image Analysis Computer vision (CV) and image processing techniques are advanced methods for biomedical image analysis with applications in lesion detection and segmentation, clustering, tracking, and identification across multiple imaging modalities (e.g., X-ray, Computed Tomography, and Functional Magnetic Resonance Imaging). The project is multidisciplinary and will expose the REU student to principles from physics, biomedical engineering, signal processing, and computer science, while gaining experience with biomedical sensors, devices, data, and algorithms. Prior to arriving on campus, the student working with Dr. Riggan will have user accounts created on the lab’s high performance computing environment and receive essential training and resources to access data effectively and safely. By the end of the first week on campus, the REU student will be introduced to project specific sensors, sensor phenomenology, and sensor data. In weeks 2-4, the student will complete a semi-automated data curation process using our AI-enabled data annotation tools and will sketch out design principles for CV algorithms, such as physical characteristics, texture, and other patterns of interests. In weeks 5-7, they will create a new CV algorithm to detect, cluster, track, or identify anomalies in biomedical imagery. Then, students will develop their data analysis plans in collaboration with graduate students. In weeks 8-10, they will finalize algorithm designs, execute their data analysis plans, and prepare and present their work at the REU symposium. |
Funding
Funding for this research program was generously provided by grants from:
NSF - National Science Foundation